Translate this page into:
Obesogenic diet-induced insulin-resistant rats develop neurobehavioral and memory deficits following bisphenol-A and cadmium co-exposure
*Corresponding author: Abdulwasiu Taiwo Lawal, Department of Anatomy, Faculty of Basic Medical Sciences, College of Health Sciences, University of Ilorin, Ilorin, Nigeria. law.abdulwasiu@gmail.com
-
Received: ,
Accepted: ,
How to cite this article: Lawal AT, Akinola OB. Obesogenic diet-induced insulin-resistant rats develop neurobehavioral and memory deficits following bisphenol-A and cadmium co-exposure. J Health Sci Res. doi: 10.25259/JHSR_43_2024
Abstract
Objectives
Obesogenic high-fat diets (HFD) have been widely implicated in the etiology of dementia-related neuropathologies through the disruption of brain insulin signaling. Likewise, exposure to environmental toxicants has been associated with various neurodegenerative symptoms attributed to their oxidative stress-mediated neurotoxicity. Thus, this study aims to investigate the effects of exposure to the environmental toxicants, bisphenol-A (BPA) and cadmium (Cd), on the exploratory instinct and spatial awareness memory of HFD-induced insulin-resistant adult Wistar rats.
Material and Methods
Twenty-five adult Wistar rats were randomly assigned to five groups (A–E; n = 5) and treated as follows: A: normal saline; B: 40 mg/kg.bw CdCl2+HFD+Suc; C: 40 mg/kg.bw BPA+HFD+Suc; D: 40 mg/kg.bw BPA+ 40 mg/kg.bw CdCl2+HFD+Suc; E: HFD+Suc, orally for 56 days. Rats were subjected to the Y-maze behavioral test for spatial awareness and learning memory, and the respective trials were recorded as measures of short-term memory and spatial awareness. On the last day of the experiment, brains were excised, the hippocampus was dissected from each group, and samples were processed for H&E histological investigation.
Results
Results from this study revealed that BPA and Cd impaired exploratory instincts and spatial awareness of insulin-resistant rats by significantly reducing their exploration of the Y-maze. The hippocampus of exposed rats also revealed neurodegenerative-like changes in histoarchitectural studies with extensive nuclear condensation.
Conclusion
Histomorphological and behavioral evidence from this study suggests that exposure to oxidative stress-inducing and insulin-signaling perturbing chemicals cause deficits in cognitive and short-term memory.
Keywords
Bisphenol-A
Cadmium
Insulin resistance
Memory deficit
Oxidative stress
INTRODUCTION
The hippocampus and hypothalamic nuclei play critical roles in the regulation of cognitive function and control of food intake, respectively, and both are influenced by insulin sensitivity in the brain.[1] As a result, disruption of either of these pathways results in neuropathologies that affect memory and cognition, which are the hippocampal functions. Obesity and diabetes are metabolic conditions that alter the highly regulated brain insulin transport,[2] including the regional pattern of insulin receptor expression and brain insulin action within the olfactory bulb, hypothalamus, hippocampus, cerebral cortex, and cerebellum.[3,4] The impairment of brain insulin signaling, that is, insulin resistance, termed “type 3 diabetes,” leads to the neuropathological hallmarks of Alzheimer’s disease (AD) through hyperphosphorylation of tau protein and β-amyloid (Aβ) plaque formation.[5] Insulin and leptin resistance are initiated through excessive caloric intake and high-fat diets (HFD) by the induction of mitochondrial dysfunction and hypothalamic endoplasmic reticulum stress.[1] Leptin, which is a protein hormone, acts together with insulin on the hypothalamus to suppress food intake and reduce body adiposity as well as on many brain areas to influence motivation, learning, memory and cognitive function, neuroprotection, reproduction, growth, metabolism, and expenditure of energy among others.[6]
Neurotoxic chemicals that include industrial additives and metals that constitute environmental pollution are significant factors in the etiological manifestation of various neurodegenerative conditions, as well as neurodevelopmental and psychiatric disorders.[7] Bisphenol A (BPA) is an endocrine-disrupting chemical and environmental pollutant,[8] and humans get exposed to these in varying concentrations and durations, which leads to oxidative stress due to the build-up of reactive oxygen species (ROS), increased lipid peroxidation, and alteration of DNA structure through nucleotide base modifications that disrupt DNA synthesis and repair pathways.[9-12]
Cadmium is a naturally occurring heavy metal present in the environment and has applications in industrial production and manufacturing.[13] The public health concern for heavy metals related to biological systems is due to their reported disruption of cellular components and morphology as well as critical biochemical and metabolic processes through the alteration of normal enzyme-mediated pathways and cellular processes[14] leading to tissue damage, uncontrolled cell division, and increased potential for tumorigenesis and apoptosis that affects normal histogenesis.[15] Likewise, cerebellar ataxia induced by environmental toxins and heavy metals has been reported to be owed to the perturbation to cerebellar cortical and purkinje neurons.[16] Arnold et al., (2014) reported that, in addition to inducing brain insulin resistance in both cerebral cortical tissue and hippocampus, HFDs produced impairment in spatial working memory by decreased T-maze alternation.[17] Although there are other underlying genetic and epigenetic permutations, such as gene polymorphism, that increase the risk of Alzheimer’s disease (AD), however, prolonged HFD consumption has been established as a major influence in the pathophysiology. A study by Del et al. (2018) highlighted the relationship between hippocampal learning or memory deficits and nutritional or endocrine inputs derived from HFDs on juvenile hippocampal morphology and neurotransmission.[18] A high-fat diet has been established as a promoter of hyperglycemia and whole-body insulin resistance and thus accepted as a valid rodent model for simulating the metabolic syndrome and its associated insulin resistance and compromised functions of the pancreatic beta cells.[19] Diets high in fats and calories are known as high-fat diets (HFDs) or Western diets (WDs), and as the rate of their consumption continues to rise globally, especially in the more industrialized nations, it has become a major source of public health concern due to its attending obesity associated with a myriad of metabolic diseases that includes type II diabetes, cardiovascular diseases, stroke, gastrointestinal, and respiratory diseases as well as several kinds of cancers.[20,21] Meanwhile, Akinola et al. (2011) linked poorly treated diabetes mellitus with neural complications and varying degrees of neurobehavioral manifestations.[22] In other words, diet-induced obesity (and insulin resistance) is linked with cognitive deficits and neurodegenerative diseases such as Alzheimer’s disease through mechanistic processes that involve the exacerbation of brain inflammation and disturbance of neuroplasticity.[20]
Insulin-dependent diabetes mellitus, also known as type I diabetes and insulin-independent diabetes mellitus (type 2 diabetes) are metabolic diseases that manifest in chronic hyperglycemia in the body. Type I diabetes is a condition that is characterized by a low or complete lack of pancreatic insulin production, while type 2, on the other hand, is propagated by insufficient insulin production, which impacts the glucose metabolic demands of the body. An imbalance in the systemic glucose-insulin dynamics precipitates abnormal glucose metabolism secondary to the development of insulin resistance. This is due to problems with glucose transport into the cells and its normal metabolism that maintains elevated glucose levels in the bloodstream, a characteristic of both type I and type II diabetes. The resulting metabolic complications, such as neuropathy, renal failure, retinopathy, cardiovascular diseases, and peripheral vascular diseases, make it a significant concern.[23,24]
We, therefore, aim to study the effects of oral exposure to bisphenol-A and cadmium on neurobehavioral profile and hippocampal dentate gyrus histomorphology in a model of insulin-resistant rats through neurobehavioral, biochemical, and histological assessment of changes in selected parameters.
MATERIAL AND METHODS
Ethical considerations
All experimental protocols and animal handling were under the guidelines of the University of Ilorin, Ilorin Ethical Review Committee (UERC) and were approved by the Postgraduate Ethical Review Committee of the University of Ilorin, Ilorin, with approval number UERC/ASN/2019/1854.
Chemicals and high-fat diet
Cadmium chloride (Kermel Chemical Reagent Co., Ltd., Tianjin, China) was obtained from Labtrade (Nig) Co., and Bisphenol A (Loba Chemie Pvt Ltd, India) was procured from Mich-Mikedenson Nig. Ltd. Other chemicals used were of analytical grade. High-fat feed was compounded at Ogo-Oluwa Livestock and Feed Mills, Ilorin, Nigeria.
Animals and experimental design
Twenty-five (25) adult male Wistar rats (Rattus norvegicus) (95–120 g) (Ogo-Oluwa Livestock and Feed Mills, Ilorin, Nigeria) were used for this study. The animals were kept in cages at the laboratory animal facility, the University of Ilorin, with a 12-h light/dark cycle under standard room temperature and humidity with liberal access to rat pellets (Ogo-Oluwa Livestock and Feed Mills, Ilorin, Nigeria) and distilled water.
Treatment plan
Rats were randomly assigned into five groups with five animals each as follows: Group A (control): free access to distilled water; Group B: daily oral 40 mg/kg CdCl2 + HFD + Suc; Group C: daily oral 40 mg/kg BPA + HFD + Suc; Group D: daily oral 40 mg/kg CdCl2 + 40 mg/kg BPA + HFD + Suc; and Group E: daily oral HFD + Suc ad libitum. The administration lasted for 56 consecutive days.[25–27]
Evaluation of body weight
Body weights of rats were recorded on arrival at the animal house and at each week of the 8-week study duration. Body weight changes were calculated as follows:
Behavioral test of cognitive function and memory
Three days to the end of the administration period, rats from all groups were taken through a training phase of five trials to familiarize them with the Y-maze paradigm. On the last day of the treatment period, the rats were subjected to the test phase of the Y-maze neurobehavioral paradigm test to assess spatial navigation memory and cognitive function. The protocol employs a “Y-shaped” wooden apparatus with three arms (labeled A¸ B, and C) that are at an angle from each other with each arm measuring 40 cm in length¸ 8cm in width, and 15 cm in height.
Y-maze procedure
Each animal was first placed in arm B facing away from the center and allowed to explore the apparatus for 8 min duration while the sequence of alternation between arms was recorded by an overhead camera. The trial began as soon as the animal was placed in the apparatus and ended at the elapse of the specified duration. Scoring was done by counting the number of entries into different arms of the apparatus, and an entry is defined as when all four paws of the animal are inside an arm. The animal was returned to the cage, and the apparatus was cleaned with alcohol between the trials.
Percentage alternation, which measures the tendency of the rat to enter a different arm from that previously visited, is then computed as follows:
Sample preparation
On the last day of administration, animals fasted overnight, final body weights were taken, arterial blood was collected from the tails, and fasting blood glucose was estimated by the glucose oxidase method using Accu-Check (Roche, Belgium) and animals were anesthetized with an intraperitoneal injection of ketamine (20 mg/kg) which is the only method of sacrifice employed. Blood samples were collected through cardiac puncture into appropriate plain bottles and left to clot at room temperature (about 23°C) for 30 min. Blood samples were subsequently centrifuged at 3000 × g for 15 min. The serum was decanted and frozen at −20°C for serum glucose and serum insulin analysis. Brain tissues were harvested and separated into right and left hemispheres. Hemispheres were dissected on a cold plate to excise the hippocampus. Hippocampal tissues were immediately fixed in 4% paraformaldehyde (Central Research Laboratories, Ilorin, Nigeria) for H&E histological protocol.
Assay for fasting serum insulin and glucose
Serum glucose was assayed using the glucose oxidase method diagnostic enzyme kit (Span Diagnostic Chemicals, India), and serum insulin levels were assayed using the Accu-Bind ELISA Microwells Insulin Test System (Monobind Inc. CA, USA) per the manufacturer’s instructions.
Estimation of insulin resistance
Insulin resistance was estimated using the homeostatic model assessment of insulin resistance (HOMA-IR) method as previously reported.[28]
Hippocampal tissue processing and photomicrography
Hippocampus tissues were fixed with 10% phosphate-buffered formaldehyde and subjected to the routine method for paraffin wax embedding to produce the required paraffin wax-embedded tissue blocks. To obtain the tissue blocks for staining, the fixed hippocampus tissues were taken through the routine tissue processing protocol for H&E. Photomicrographs were taken with an Axiocam ERc 5s camera attached to a Carl Zeiss AX10 microscope and analyzed using the Zen Core 3.5 software.
Data analysis
GraphPad Prism software version 9 (GraphPad Software, Inc., San Diego, CA, USA) was used to analyze data from neurobehavioral and biochemical assays, and one-way analysis of variance (ANOVA) was used to compare differences in means, followed by Tukey’s multiple comparison tests where necessary. All data are presented as mean ± SEM, and p<0.05 is considered statistically significant.
RESULTS
High fat diets–induced insulin-resistant rats show relatively higher weight changes
Table 1 shows weight increase, and the percentage change in body weight was significantly higher in the HFD+Suc (116.0±1.8, 29.96±1.13) and BPA+HFD+Suc (109.4±1.6, 26.12±1.12) groups. Meanwhile, Cd seems to prevent much weight gain as the groups exposed to the chemical recorded the lowest mean weight and percentage increase in weight, Cd+BPA+HFD+Suc (80.2±0.7, 3.18±0.83) and Cd+HFD+Suc (82.2±1.9, 22.22±2.11), respectively.
Groups | Initial weight (g) | Final weight (g) | Weight difference (%) |
---|---|---|---|
Ngtv Ctrl | 73.4±1.8 | 98.4±2.4 | 25.14±3.17 |
Cd+HFD+Suc | 63.3±1.4 | 82.2±1.9 | 22.22±2.11 |
BPA+HFD+Suc | 80.8±1.3 | 109.4±1.6* | 26.12±1.12 |
Cd+BPA+HFD+Suc | 78.2±1.3 | 80.2±0.7 | 3.18±0.83 |
HFD+Suc | 81.8±1.6 | 116.0±1.8* | 29.96±1.13 |
Values are expressed as mean±SEM with the level of significance shown as *(P<0.05). SEM: Standard error of mean, Ngtv Ctrl: Negative control, Cd:Cadmium, HFD: High-fat diet, BPA: Bisphenol-A, Suc: Sucrose.
Exposure to high-fat diet and sucrose resulted in significantly higher HOMA-IR scores in exposed rats
Figure 1 below shows significantly higher (p<0.05) HOMA-IR scores of HFD-exposed groups compared to the negative control. This result suggests decreased sensitivity of their tissues to the glucose regulatory effects of insulin.
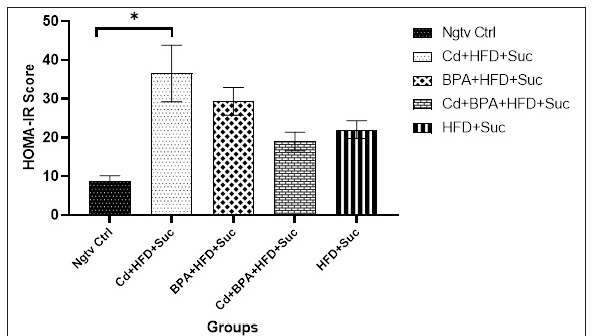
- HOMA IR score across all rat groups exposed to distilled water (Ngtv Ctrl), Cd+HFD+Suc, BPA+HFD+Suc, Cd+BPA+HFD+Suc, HFD+Suc after 56 days of exposure. * significant (p<0.05) difference when compared to Ngtv Ctrl. Ngtv Ctrl: Negative control, Cd:Cadmium, HFD: High-fat diet, BPA: Bisphenol-A, Suc: Sucrose, HOMA-IR: Homeostasis model assessment of insulin resistance.
BPA and Cd exposure induced motor-related and memory deficits in insulin-resistant rats
Table 2 and Figure 2 below show the results of the Y-maze behavioral paradigm that tests the short-term memory and spatial awareness or alternation of the experimental animals.
Groups | Spatial alternation (%) |
---|---|
Ngtv Ctrl | 31.30±13.40 |
Cd+HFD+Suc | 21.40±5.80* |
BPA+HFD+Suc | 17.00±9.10* |
Cd+BPA+HFD+Suc | 3.00±3.00* |
HFD+Suc | 33.20±3.50 |
Values are expressed as mean±SEM with the level of significance shown as *(P<0.05). SEM: Standard error of mean, Ngtv Ctrl: Negative control, Cd: Cadmium, HFD: High-fat diet, BPA: Bisphenol-A, Suc: Sucrose.
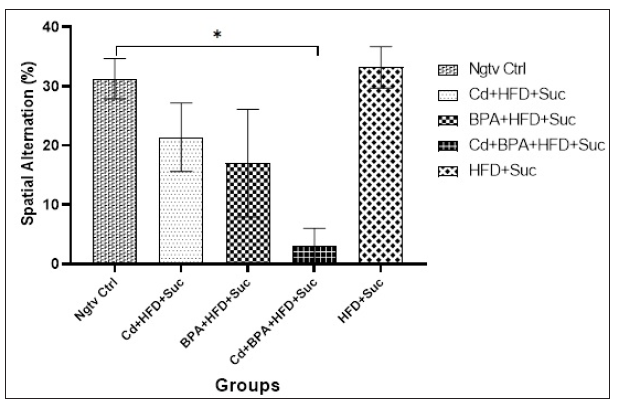
- Arm alternation frequency scores across rat groups exposed to distilled water (Ngtv Ctrl), Cd+HFD+Suc, BPA+HFD+Suc, Cd+BPA+HFD+Suc, HFD+Suc after 56 days of exposure. * significant (p<0.05) difference when compared to Ngtv Ctrl. Ngtv Ctrl: Negative control, Cd: Cadmium, HFD: High-fat diet, BPA: Bisphenol-A, Suc: Sucrose.
Hippocampus reveals disruptions in histoarchitecture and neuronal morphology following exposure to BPA and Cd
Cellular composition and staining intensities appear to be rather consistent and even across the groups. However, groups exposed to obesogenic diets and environmental insults (BPA and Cd) present with more deeply pigmented nuclei with poor delineation in the cells of the dentate gyrus (DG) and CA regions with large halo-spaces compared to the negative control group [Figure 3]. These observations suggest the disintegration of nuclear material (karyolysis), breakdown of the nuclear membrane (karyorrhexis), and granule cell loss, which are consistent with cellular degeneration.
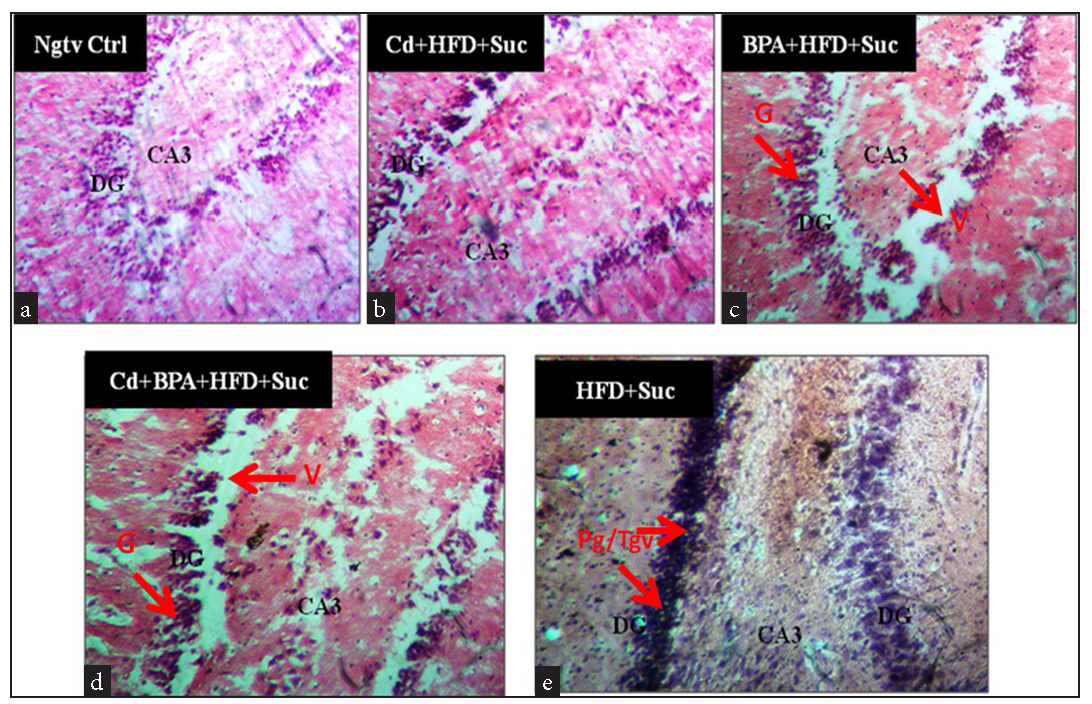
- Representative light micrograph of the dentate gyrus of all groups exposed for 56 days. (a) Ngtv Ctrl (x100), (b) Cd+HFD+Suc (x200), (c) BPA+HFD+Suc (x200), (d) Cd+BPA+HFD+Suc (x200) and (e) HFD+Suc (x200).Stain: H&E. BV: Blood vessel, G: Granule cell, V: Vacuolation, Nbv: Necrotic blood vessel, Cl: Clumping, Tg: Tangling, Pg: Deeply pigmented cells, H&E: Hematoxylin and eosin, DG: Dentate gyrus, CA3: CA3 region, Ngtv ctrl: Negative control group, Cd: Cadmium, HFD: High-fat diet, Suc: Sucrose drink, BPA: Bisphenol-A, Red arrows: pointing to the cells/features of interest.
DISCUSSION
Industrialization has contributed to the increased exposure of humans to heavy metals such as cadmium, aluminum, lead, arsenic, and others.[29] Through primarily oxidative stress and mitochondrial dysfunction, these metals induce various levels of toxicities with manifestations ranging from motor to cognitive impairments.[30–32]
Results from this study indicate that exposure to HFDs and sucrose drinks precipitate insulin resistance in animals, which leads to higher serum insulin and serum glucose levels in the exposed groups compared to the control. Serum glucose and serum insulin were the insulin-glucose homeostasis markers analyzed, and the reported results suggest that combined exposure to HFDs and sucrose results in high serum glucose and insulin levels. This observation is further buttressed by the reported higher score for assessment of insulin resistance (HOMA-IR) in the HFDs and sucrose-treated groups of the current study. A similar study by Mohammed et al. (2022) reported that insulin resistance prolonged escape latency in diabetic rats.[33] On exploratory behavior, the Y-maze test revealed that the environmental toxicants (bisphenol A and cadmium) were capable of producing behavioral deficits in rat models, both alone and on coexposure, as observed in the different treated groups. The Y-maze paradigm was deliberately used alone in this study to further demonstrate this paradigm as a sufficient stand-alone assay for short-term spatial working memory neurobehavioral studies. Histopathological examination of the hippocampal dentate gyrus reveals neuronal loss and nuclear condensation with tangling and deeply pigmented cells of the dentate gyrus. Similarly, cytopathological analysis from a study reported a decreased number of pyramidal cells in the CA1 region coupled with damages to mitochondria and endoplasmic reticulum of hippocampal cells.[34] Imam et al. (2022) also reported global cerebellar neurodegenerative changes characterized by numerous perineural spaces and reductions in neural density indicative of cellular shrinkage following aluminum chloride exposure.[30] The reported cellular damages characterized by neuronal loss and condensation of neuronal nuclei as observed in the environmental toxicants treated groups in this study provide evidence of the deleterious effects of these environmental toxicants and their culpability in the cognitive and exploratory memory deficits as observed in the model rats.
CONCLUSION
Reports from this work lend further credence to previous studies on the effects of environmental toxicants on cognitive memory and exploratory skills of rat models, as well as the neuroendocrine-disrupting effects of HFDs. Overall, we demonstrated that isolated or coexposure to cadmium chloride and BPA in HFD-induced insulin-resistant rats precipitated hippocampal cortical lesions that could progress to neurodegenerative changes with the attending memory and cognitive deficits.
Ethical approval
The research/study was approved by the Institutional Review Board at the University of Ilorin, Ilorin, Nigeria, number UERC/ASN/2019/1854, dated September, 2019.
Declaration of patient consent
Patient consent is not required as there are no patients in this study.
Financial support and sponsorship
Nil.
Conflicts of interest
There are no conflicts of interest.
Use of artificial intelligence (AI)–assisted technology for manuscript preparation
The authors confirm that there was no use of artificial intelligence (AI)-assisted technology for assisting in the writing or editing of the manuscript and no images were manipulated using AI.
REFERENCES
- Insulin resistance in brain and possible therapeutic approaches. Curr Vasc Pharmacol. 2014;12:553-64.
- [CrossRef] [PubMed] [Google Scholar]
- Differential permeability of the blood-brain barrier to two pancreatic peptides: Insulin and amylin. Peptides. 1998;19:883-9.
- [CrossRef] [PubMed] [Google Scholar]
- Insulin receptors are widely distributed in the central nervous system of the rat. Nature. 1978;272:827-9.
- [CrossRef] [PubMed] [Google Scholar]
- Distribution of insulin receptor-like immunoreactivity in the rat forebrain. Neuroscience. 1989;31:143-57.
- [CrossRef] [PubMed] [Google Scholar]
- Insulin action in the brain regulates both central and peripheral functions. Am J Physiol Endocrinol Metab. 2021;321:E156-63.
- [CrossRef] [PubMed] [PubMed Central] [Google Scholar]
- Leptin signaling in brain: A link between nutrition and cognition? Biochim Biophys Acta. 2009;1792:401-8.
- [CrossRef] [PubMed] [PubMed Central] [Google Scholar]
- Environmental Toxins and Brain: Life on Earth is in Danger. Ann Indian Acad Neurol. 2022;25:S15-S21.
- [CrossRef] [PubMed] [PubMed Central] [Google Scholar]
- Comparative study of environmental pollutants bisphenol A and bisphenol S on sexual differentiation of anteroventral periventricular nucleus and spermatogenesis. Reprod Biol Endocrinol. 2019;17:53.
- [CrossRef] [PubMed] [PubMed Central] [Google Scholar]
- Bisphenol A as an environmental pollutant with dual genotoxic and DNA-protective effects. Neuro Endocrinol Lett. 2018;39:294-8.
- [PubMed] [Google Scholar]
- A study on environmental bisphenol A pollution in plastics industry areas. Water Air Soil Pollut. 2017;228:1-9.
- [CrossRef] [PubMed] [PubMed Central] [Google Scholar]
- Analysis of effects of a new environmental pollutant, bisphenol A, on antioxidant systems in soybean roots at different growth stages. Sci Rep. 2016;6:23782.
- [CrossRef] [PubMed] [PubMed Central] [Google Scholar]
- Gene expression changes in human lung cells exposed to arsenic, chromium, nickel or vanadium indicate the first steps in cancer. Metallomics. 2012;4:784-93.
- [CrossRef] [PubMed] [PubMed Central] [Google Scholar]
- Heavy metal toxicity and the environment. Exp Suppl. 2012;101:133-64.
- [CrossRef] [PubMed] [PubMed Central] [Google Scholar]
- Molecular mechanisms of metal toxicity and carcinogenesis. Mol Cell Biochem. 2001;222:3-9.
- [CrossRef] [PubMed] [Google Scholar]
- Toxic agents causing cerebellar ataxias. Handb Clin Neurol. 2012;103:201-13.
- [CrossRef] [PubMed] [Google Scholar]
- High fat diet produces brain insulin resistance, synaptodendritic abnormalities and altered behavior in mice. Neurobiol Dis. 2014;67:79-87.
- [CrossRef] [PubMed] [PubMed Central] [Google Scholar]
- Influence of high-fat diets consumed during the juvenile period on hippocampal morphology and function. Front Cell Neurosci. 2018;12:439.
- [CrossRef] [PubMed] [PubMed Central] [Google Scholar]
- Defining high-fat-diet rat models: Metabolic and molecular effects of different fat types. J Mol Endocrinol. 2006;36:485-501.
- [CrossRef] [PubMed] [Google Scholar]
- Long-term diet-induced obesity does not lead to learning and memory impairment in adult mice. PLoS One. 2021;16:e0257921.
- [CrossRef] [PubMed] [PubMed Central] [Google Scholar]
- Cognitive impairment following high fat diet consumption is associated with brain inflammation. J Neuroimmunol. 2010;219:25-32.
- [CrossRef] [PubMed] [PubMed Central] [Google Scholar]
- Diabetes-induced prefrontal Nissl substance deficit and the effects of neem-bitter leaf extract treatment. Int J Morphology. 2011;29:850-6.
- [CrossRef] [Google Scholar]
- Cost and cost-effectiveness of mHealth interventions for the prevention and control of type 2 diabetes mellitus: A protocol for a systematic review. BMJ Open. 2019;9:e027490.
- [CrossRef] [PubMed] [PubMed Central] [Google Scholar]
- Distribution and cellular localization of KCC2 in the Ferret Neocortex. Dev Neurosci. 2018;40:39-53.
- [CrossRef] [PubMed] [Google Scholar]
- Guidelines on dosage calculation and stock solution preparation in experimental animals’ studies. J Natural Sci. 2014;4
- [Google Scholar]
- Toxicity and distribution of cadmium administered to rats at sublethal doses. Toxicol Appl Pharmacol. 1977;41:667-80.
- [CrossRef] [PubMed] [Google Scholar]
- Homeostasis model assessment: Insulin resistance and beta-cell function from fasting plasma glucose and insulin concentrations in man. Diabetologia. 1985;28:412-9.
- [CrossRef] [PubMed] [Google Scholar]
- “Nano-killers”. Aluminium toxicity in the human body. Research Association for Interdisciplinary Studies 2019;83–90. (Balusescu GM, & Horhogea M (2019). “Nano-Killers. Aluminium toxicity in the Human Body”, Proceedings of the 15th International RAIS Conference, November 6–7, 2019. Research Association for Interdisciplinary Studies.) Available from: https://doi.org/10.5281/zenodo.3550051
- Nigella sativa oil ingestion mitigates aluminium chloride induced cerebellar oxidative, neurogenic damages and impaired motor functions in rats. Anatomy J Africa. 2022;11:2109-21.
- [Google Scholar]
- Lasting metabolic effect of a high-fructose diet on global cerebral ischemia. Nutr Neurosci. 2022;25:1159-72.
- [CrossRef] [PubMed] [Google Scholar]
- Effects of bisphenol A on oxidative stress in the rat brain. Antioxidants (Basel). 2020;9:240.
- [CrossRef] [PubMed] [PubMed Central] [Google Scholar]
- The effects of flavonoids in curcumin on neurobehavioural deficits in insulin-resistant rats. J Neurobehavioural Sciences. 2022;9:51-7.
- [Google Scholar]
- Obesity, diabetes and cognitive deficit: The framingham heart study. Neurobiology of Aging 2005:111-16.
- [Google Scholar]