Translate this page into:
Invertebrate Models to Assess Environmental Exposures and Toxicity of Silver Nanoparticles
*Email: zorawarsinghs@rediffmail.com
Abstract
Over the past few decades, nanoparticles of noble metals such as silver and gold have been found to demonstrate significantly distinct physical, chemical, and biological properties from their bulk counterparts. Nano-sized particles of less than 100 nm in diameter are attracting the scientific community for the wide range of new applications in various fields including biophysics, material and medical sciences. As manufacturing of nano-sized particles is increasing, their dissemination in natural environments is also at an increase. Finding the adverse effects of such nano-sized particles found in the environment on different faunal species is thus of utmost importance. In the present paper, toxic effects of silver nanoparticles on different invertebrate models have been presented so as to have a view about the type of the damage posed by them and the efficacy of using invertebrate models for such assessments.
Keywords
Environment
Exposure
Genotoxicity
Invertebrate Models
Oxidative Stress
Silver Nanoparticles
Toxicity
Introduction
Nano-sized particles are near atomic scale size and have at least one dimension in the range of 1-100 nm. Relatively little is known about the behavior and toxicity of nanoparticles in the environment. Nano-sized particles are attracting the scientific community for their wide range of applications in various fields1. Nanoparticles can exhibit properties that differ markedly from those of bulk materials, due to their small particle dimension, high surface area and quantum confinements. Silver nanoparticles (SNPs) are likely to enter the aquatic ecosystems because of their multiple applications and pose a health concern for humans and aquatic species2. Although SNPs are increasingly used in various consumer products and produced at industrial scale3, information on harmful effects of nanosilver to environmentally relevant organisms is still scarce. In this paper, a review of toxicological aspects of SNPs has been presented on different invertebrate models.
Invertebrate Models used for Assessing Toxicological Aspects of SNPs
Daphnia magna
Daphnia magna, a planktonic crustacean (Figure 1) has been used by different researchers as an invertebrate model for assessing toxic aspects of SNPs1,3-6. Study of the effects of filtration and capping agents and absence or presence of food was conducted on the toxicity of SNPs to Daphnia magna7. Study was done by exposing them with silver nitrate solution and SNP suspensions including both commercially available SNPs (coated & uncoated) and laboratory synthesized SNP’s (coated with coffee or citrate). Toxicity of filtered and unfiltered suspensions was compared and effect on addition of food was also examined. Dose response curves were generated and median Lethal Concentration (LC50) values calculated. The LC50 values for unfiltered particles were found to be 1.1 ± 0.1µg/L - AgNO3 ; 1.0 ± 0.1-coffee coated; 1.1 ± 0.2-citrate coated; 16.7 ± 2.4 Sigma Aldrich Ag-nanoparticles (SA) uncoated; 31.5 ± 8.1 SA coated. LC50 values for the filtered particles were 0.7 ± 0.1 -AgNO3; 1.4 ± 0.1 -SA uncoated; 4.4 ± 1.4 -SA coated. Similarly8 studied the comparison of acute and chronic toxicity of SNPs and silver nitrate in Daphnia magna. When D. magna was exposed to SNPs for toxicity test (48 hrs.), they observed no mortality but when they exposed D. magna with SNPs for chronic exposure (21 days), it was observed that water-borne AgNO3 inhibited the growth and reproduction in D. magna

Studied4 the toxicity of various SNP’s compared to silver ion in D. magna and found that it caused swimming abnormality in D. magna and the toxicity of silver species is dose and composition dependent. The colloidal SNPs and AgNO3 were almost similar in terms of mortality. On the same line studied9 the importance of surface coatings on SNP’s toxicity in D. magna. The study revealed the effect of 3 surface coatings and observed difference in mortality among three coating exposures for 48 hrs. LC50 was found dependent on the characteristics of nano-particles including particle size and surface coating. Studied1 the toxicity of 2 types of silver nano-particles to D. magna and Thamnocephalus platyurus. In this study, the toxicity of collargal (protein-coated nanosilver) and AgNO3 was analyzed and both were found to be highly toxic to both crustaceans. EC50 values in artificial freshwater were 15-17 ppb for D. magna and 20-27 ppb for T. platyurus whereas natural water mitigated toxic effect up to 8 folds as compared to artificial fresh water. The toxicity of SNPs in all test media was up to 10-fold lower than that of soluble silver salt, AgNO3. Studied10 the SNP’s and AgNO3 induced high toxicity to Pseudokirchneriella subcapitata, Daphnia magna and Danio rerio. In this study, the SNPs were characterised to investigate the aggregation and agglomeration behaviour of SNPs under different media, chemical composition and test period. The results revealed that the toxicity of both AgNO3 and SNPs differ significantly based on the test species. They found no difference in toxicity for algae, a small difference for zebra fish and a major difference in toxicity for D. magna.
Caenorhabditis elegans
Apart from D. magna, various other invertebrate models have also been explored for assessing toxicity of SNPs including Caenorhabditis elegans (Figure 2)11-13. Studied11 SNP and silver ion toxicity in the nematode, C. elegans and reported that Ag nanotoxicity is dependent not only on dissolution of Ag ion but also on particle specific effects. Similarly13 studied the intracellular uptake and associated toxicity of SNPs in C. elegans. In this study, they used wild type of C. elegans and the strain was expected to be sensitive to oxidative stress, genotoxins and metals. They observed growth inhibition by all tested SNPs at low concentration. They also describe a modified growth assay that permits differentiation between direct growth inhibitory effects and indirect inhibition mediated by toxicity to the food source. Studied12 the oxidative stress related PMK-1 p38 MAPK activation as a mechanism for toxicity of SNPs to reproduction in the C. elegans and they concluded that oxidative stress is an important mechanism of SNP-induced reproduction toxicity in C. elegans, and that PMK-1 p38 MAPK plays an important role in it.
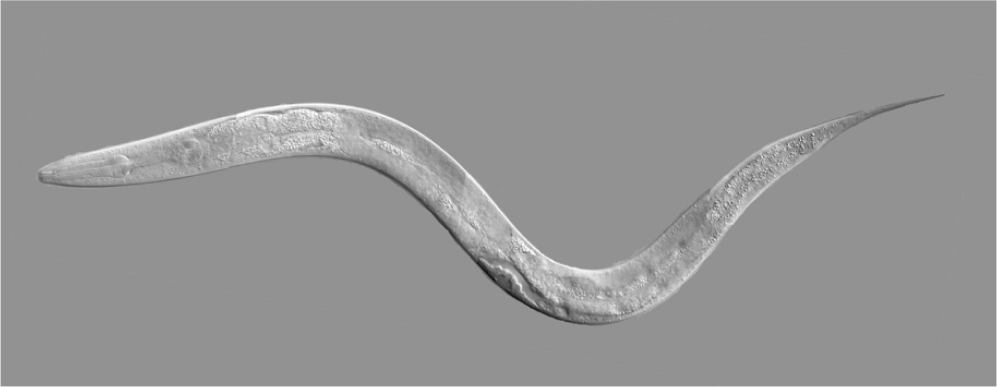
Drosophila melanogaster
Studied14 the acute and chronic toxicity effect of SNPs on Drosophila melanogaster. SNPs were prepared in the form of solid dispersion using micro-encapsulation method. The acute toxic effect of SNPs was observed for the silver concentration of 20 mg/L. At this silver concentration, 50% of the tested flies were unable to leave the pupae, and they did not finish their developmental cycle. Chronic toxicity of SNPs was assessed by a long-term exposure of overall eight filial generations of Drosophila melanogaster to SNPs. The long-term exposure to silver NPs influenced the fertility of Drosophila during the first three filial generations, nevertheless the fecundity of flies in subsequent generations consequently increased up to the level of the flies from the control sample due to the adaptability of flies to the SNPs exposure (Figure 3).
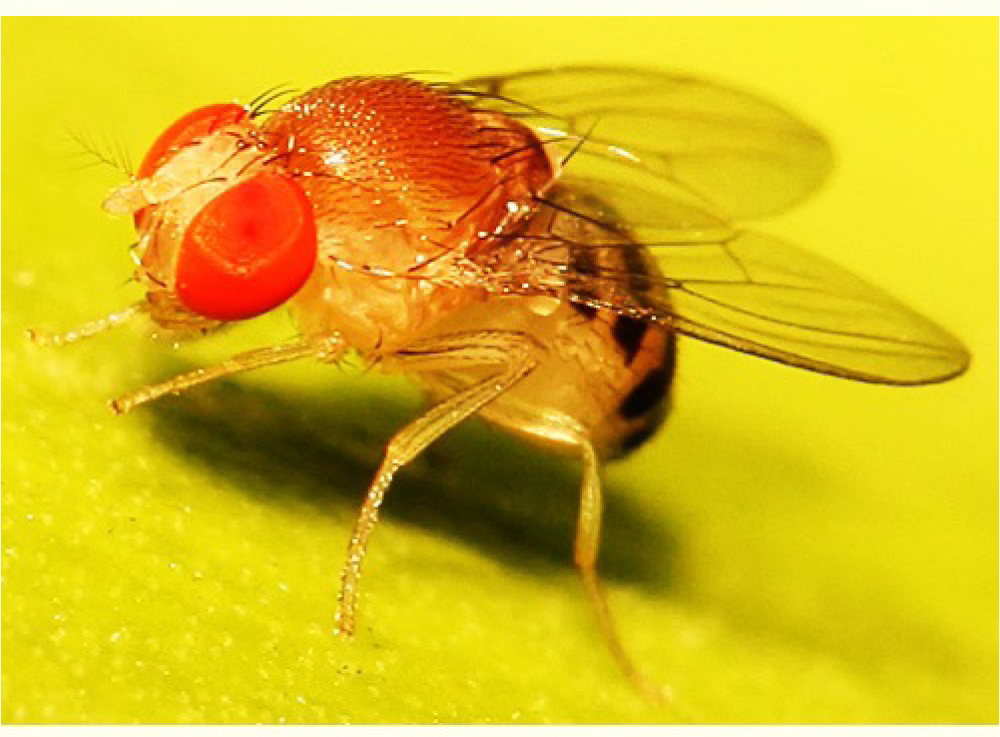
Hydra
Hydra has been used in different studies as a successful invertebrate model. Activated Carbon from Pyrolysed sugarcane Bagasse (ACPB) presented pore size ranges from 1.0 to 3.5nm which was successfully loaded with silver nanoparticles of diameter around 35nm. These loaded nanoparticles (ACPB-AgNP) showed environmental risks, with toxic effect to the aquatic organism Hydra attenuata (LC50 value of 1.94mg/L)15 (Figure 4).
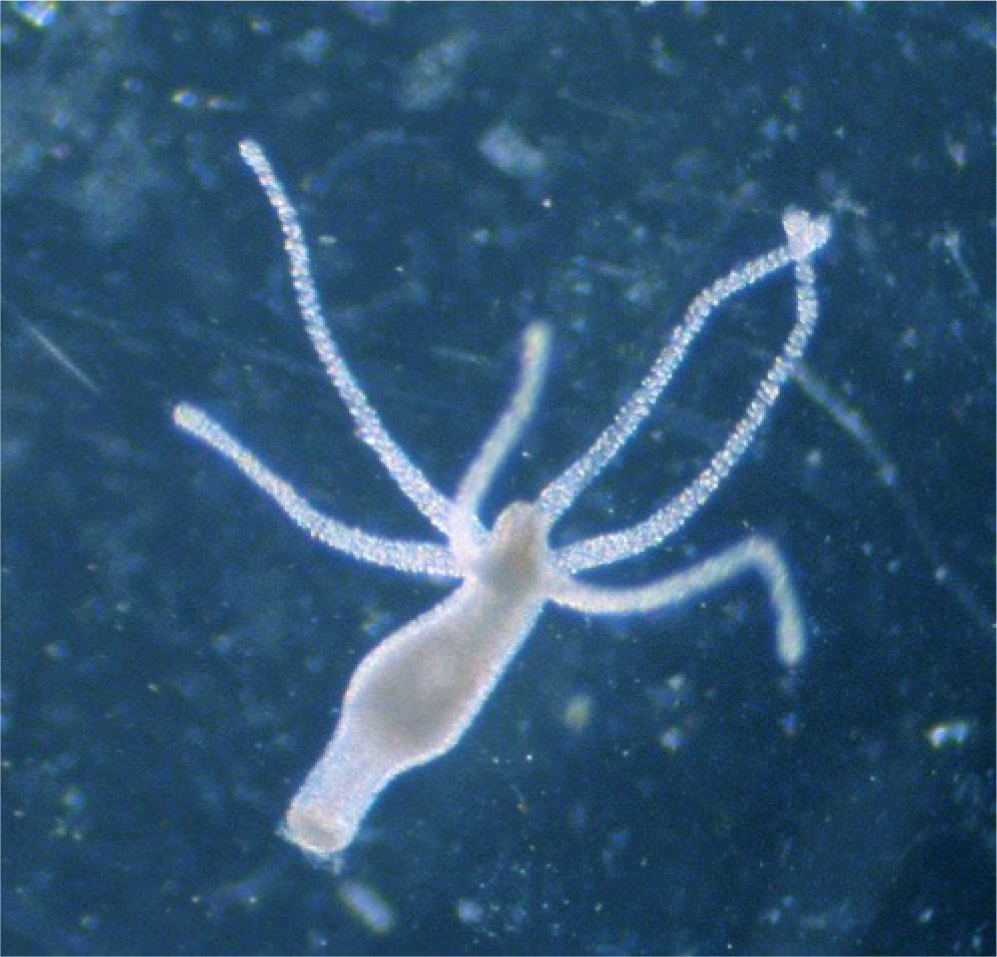
Snails
Silver is one of the most toxic metals to freshwater aquatic organisms16. Molluscs have always been used for assessing environmental stresses and toxicity parameters. Snails of different genera have been found to be employed to assess the toxic effects of SNPs also17-22. Study16 the induction of apoptosis and DNA damage by SNPs in snail Lymnea luteola. Three concentrations of SNPs were selected, the concentration I (4 mug/l), concentration II (12 mug/l), and the concentration III (24 mug/l).Induction of oxidative stress in snail hemolymph was observed by a decrease in reduced glutathione (GSH) and Glutathione S-Transferase (GST) levels at different concentration of SNPs, and on the other hand, malondialdehyde (MDA) levels increased at lower concentrations but decreased in higher concentration of SNPs. DNA damage scores increased with the exposure levels of SNPs, and dose- and time-dependent effects were observed16. In another study2, L. luteola was used to investigate the acute toxicity and genotoxicity of SNPs in a static-renewal system for 96 h. SNPs caused molluscicidal activity in L. luteola, with 96-h median lethal concentrations (LC50: 48.10 µg L-1). It was observed that SNPs (36 µg L-1) elicited a significant (p<0.01) reduction in glutathione, glutathione-s-transferase and glutathione peroxidase with a concomitant increase in malondialde-hyde level and catalase in digestive gland of L. luteola.
Another snail Physa acuta was chosen to evaluate the potential deleterious effects of SNPs and their counterpart AgNO3, through water-only exposures23. 2 media were tested: Artificial Pond Water (APW) and modified APW (adapted by removing calcium chloride).Chloride was found playing an important role in toxicity, most likely by complexation with silver ions, which would reduce the bioavailability, uptake, and toxicity of silver. Chronic tests showed that hatching success was more sensitive to silver in the ionic form than in the particulate form23.
Peringia ulvae were exposed to 150 µg Ag L-1 added as citrate capped SNPs or aqueous Ag (AgNO3) in combination with inhibitor treatment24. No inhibitor treatment completely eliminated the uptake of Ag in either aqueous or NP form, but all inhibitor treatments, except phenamil, significantly reduced the uptake of Ag presented as Ag NPs. Clathrin- and caveolae-mediated endocytosis appear to be mechanisms exploited by SNPs, with the latter pathway only active at 24 h24. Another study evaluated whether water chemistry affects the dietary uptake and toxicity of SNPs by the freshwater snail Lymnaea stagnalis25. The study characterized the effects of water hardness and humic acids on the bioaccumulation and toxicity of SNPs coated with PolyVinyl Pyrrolidone (PVP) to the freshwater snail L. stagnalis after dietary exposures. Results indicated that bioaccumulation and toxicity of Ag from PVP-SNPs ingested with food are not affected by water hardness and by humic acids, although both could affect interactions with the biological membrane and trigger nanoparticle transformations25. Similarly, another study characterized the bioavailability of Ag from AgNO3 and from SNPs capped with polyvinylpyrrolidone (PVP SNP) and thiolated polyethylene glycol (PEG SNP) in the freshwater snail, L. stagnalis, after short waterborne exposures. But in this study, results showed that water hardness, SNP capping agents, and metal speciation affected the uptake rate of Ag from SNPs26 (Figure 5).
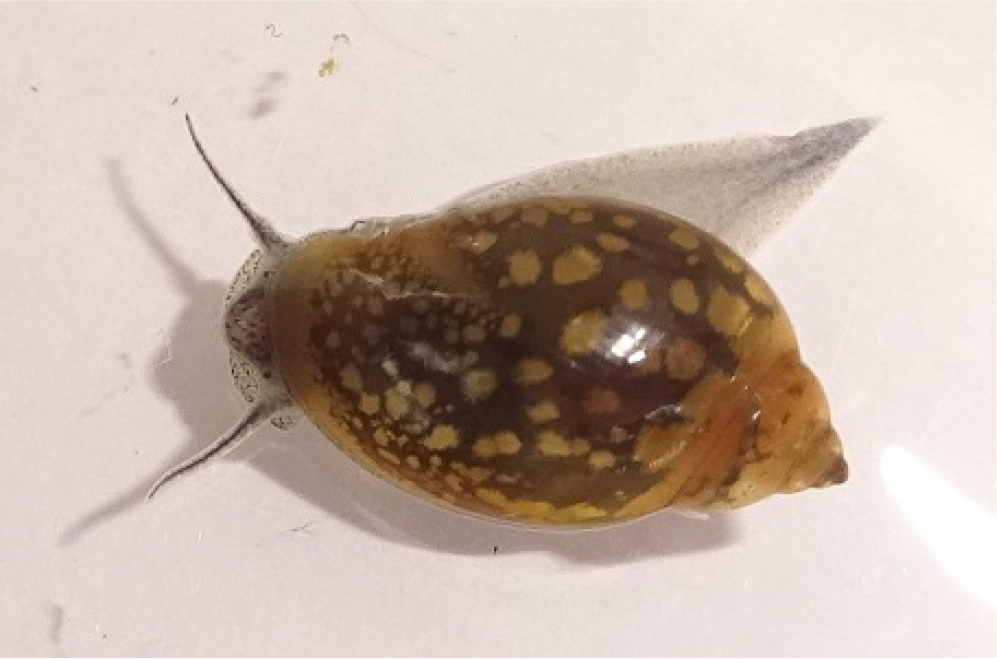
Conclusion
Silver nanoparticles are important because of their multiple applications in different fields of science. These nanoparticles are likely to enter the aquatic ecosystems and pose a health concern for humans and aquatic species. This paper emphasized on the use of different invertebrate models in various researches so as to put forward the direct or indirect impact of silver nanoparticles. We can see that a variety of invertebrates including coelenterates, roundworms, crustaceans and molluscs have been used. Further studies are recommended to compile the full toxic profile of silver nanoparticles present in the ecosystem.
References
- Toxicity of two types of silver nanoparticles to aquatic crustaceans Daphnia magna and Thamnocephalus platyurus. Environ. Sci. Pollut. Res. Int. 2013;20(5):3456-63.
- [CrossRef] [PubMed] [Google Scholar]
- Sensitivity of freshwater pulmonate snail Lymnaea luteola, to silver nanoparticles. Chemosphere. 2014;104:134-40.
- [CrossRef] [PubMed] [Google Scholar]
- Aggregation and dispersion of silver nanoparticles in exposure media for aquatic toxicity tests. J. Chromatogr. A. 2011;1218(27):4226-33.
- [CrossRef] [PubMed] [Google Scholar]
- Toxicity of various silver nanoparticles compared to silver ions in Daphnia magna. J. Nanobiotechnology. 2012;10:14.
- [CrossRef] [PubMed] [Google Scholar]
- Influence of Suwannee River humic acid on particle properties and toxicity of silver nanoparticles. Chemosphere. 2012;89(1):96-101.
- [CrossRef] [PubMed] [Google Scholar]
- Impact of organic carbon on the stability and toxicity of fresh and stored silver nanoparticles. Environ. Sci. Technol. 2012;46(19):10772-80.
- [CrossRef] [PubMed] [Google Scholar]
- Effects from filtration, capping agents, and presence/absence of food on the toxicity of silver nanoparticles to Daphnia magna. Environ. Toxicol. Chem. 2010;29(12):2742-50.
- [CrossRef] [PubMed] [Google Scholar]
- Comparison of acute and chronic toxicity of silver nanoparticles and silver nitrate to Daphnia magna. Environ. Toxicol. Chem. 2011;30(4):885-92.
- [CrossRef] [PubMed] [Google Scholar]
- Importance of surface coatings and soluble silver in silver nanoparticles toxicity to Daphnia magna. Nanotoxicology. 2012;6(4):361-70.
- [CrossRef] [PubMed] [Google Scholar]
- Silver nanoparticles and silver nitrate induce high toxicity to Pseudokirchneriella subcapitata, Daphnia magna and Danio rerio. Sci. Total Environ. 2013;466-467C:232-41.
- [CrossRef] [PubMed] [Google Scholar]
- Hypoxia inducible factor-1 (HIF-1)-flavin containing monooxygenase-2 (FMO-2) signaling acts in silver nanoparticles and silver ion toxicity in the nematode, Caenorhabditis elegans. Toxicol. Appl. Pharmacol. 2013;270(2):106-13.
- [CrossRef] [PubMed] [Google Scholar]
- Oxidative stress-related PMK-1 P38 MAPK activation as a mechanism for toxicity of silver nanoparticles to reproduction in the nematode Caenorhabditis elegans. Environ. Toxicol. Chem. 2012;31(3):585-92.
- [CrossRef] [PubMed] [Google Scholar]
- Intracellular uptake and associated toxicity of silver nanoparticles in Caenorhabditis elegans. Aquat. Toxicol. 2010;100(2):140-50.
- [CrossRef] [PubMed] [Google Scholar]
- Acute and chronic toxicity effects of silver NanoParticles (NPs) on Drosophila melanogaster, Environ. Sci. Technol. 2011;45(11):4974-79.
- [CrossRef] [PubMed] [Google Scholar]
- Activated carbon from pyrolysed sugarcane bagasse: Silver nanoparticle modification and ecotoxicity assessment. Sci. Total Environ. 2016;565:833-40.
- [CrossRef] [PubMed] [Google Scholar]
- Oxidative stress-mediated apoptosis and genotoxicity induced by silver nanoparticles in freshwater snail Lymnaea luteola. Biol. Trace Elem. Res. 2014;162(1-3):333-41.
- [CrossRef] [PubMed] [Google Scholar]
- Effects of silver nanoparticles on the freshwater snail Physaacuta: The role of test media and snails’ life cycle stage. Environ. Toxicol. Chem. 2017;36(1):243-53.
- [CrossRef] [PubMed] [Google Scholar]
- Influence of hardness on the bioavailability of silver to a freshwater snail after waterborne exposure to silver nitrate and silver nanoparticles. Nanotoxicology. 2015;9(7):918-27.
- [CrossRef] [PubMed] [Google Scholar]
- Oxidative stress-mediated apoptosis and genotoxicity induced by silver nanoparticles in freshwater snail Lymnaea luteola. Biol. Trace Elem. Res. 2014;162(1-3):333-41.
- [CrossRef] [PubMed] [Google Scholar]
- Inhibition of potential uptake pathways for silver nanoparticles in the estuarine snail Peringiaulvae. Nanotoxicology. 2015;9(4):493-501.
- [CrossRef] [PubMed] [Google Scholar]
- Does water chemistry affect the dietary uptake and toxicity of silver nanoparticles by the freshwater snail Lymnaea stagnalis? Environ. Pollut. 2014;189:87-91.
- [CrossRef] [PubMed] [Google Scholar]
- Sensitivity of freshwater pulmonate snail Lymnaea luteola, to silver nanoparticles. Chemosphere. 2014;104:134-40.
- [CrossRef] [PubMed] [Google Scholar]
- Effects of silver nanoparticles on the freshwater snail Physaacuta: The role of test media and snails’ life cycle stage. Environ. Toxicol. Chem. 2017;36(1):243-53.
- [CrossRef] [PubMed] [Google Scholar]
- Inhibition of potential uptake pathways for silver nanoparticles in the estuarine snail Peringi aulvae. Nanotoxicology. 2015;9(4):493-501.
- [CrossRef] [PubMed] [Google Scholar]
- Does water chemistry affect the dietary uptake and toxicity of silver nanoparticles by the freshwater snail Lymnaea stagnalis? Environ. Pollut. 2014;189:87-91.
- [CrossRef] [PubMed] [Google Scholar]
- Influence of hardness on the bioavailability of silver to a freshwater snail after waterborne exposure to silver nitrate and silver nanoparticles. Nanotoxicology. 2015;9(7):918-27.
- [CrossRef] [PubMed] [Google Scholar]